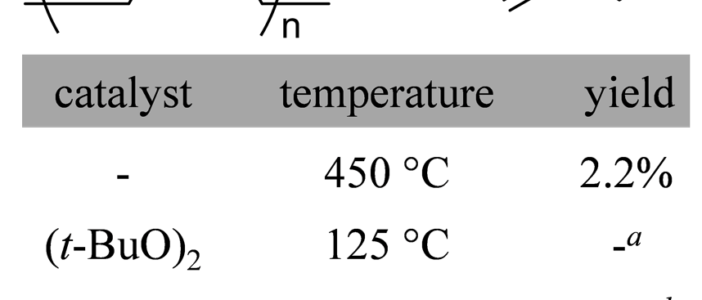
Extraction and Depolymerization of Natural Rubber from Common Milkweed
By Kevin Jack '16
CHEM 430: Advanced Organic Chemistry
The writing intensive Advanced Organic Chemistry course focuses on the development of disciplinary, technical writing skills. The assignment represented here required students to write a research article in the format of the Journal of Organic Chemistry based on the experiments they performed during the lab section of this course. While the typical style for submission to this journal is technical I thought Kevin was able to write this piece in a way that was accessible to a lay audience and still met the high standard of technical writing and experimental results
-Jay Wackerly
Abstract
Isoprene is a common biologically produced hydrocarbon and the monomer of natural rubber. Most natural rubber is extracted from Hevea brasiliensis, a tree native to South America. Here we describe attempts to recover isoprene from Asclepias syriaca, common milkweed, which is native to and abundant in Iowa. Natural rubber was extracted from milkweed through a series of liquid solid extractions. Depolymerization of isoprene from natural rubber was attempted by thermal, peroxide catalyzed, and transition metal catalyzed methods. Characterization of the recovered isoprene was accomplished by 1H NMR and GC analysis.
Introduction
Natural rubber (NR) is a polymer of isoprene produced in many plants, most notably the rubber tree, Hevea Brasiliensis. Around 98% of the NR produced comes from this tree.1 However, there have been numerous attempts at the isolation of NR from plants domestic to North America.2,3,4,5 Early in the 20th century interest in finding a domestic source for natural rubber stemmed from its utility as one of the most important polymers in the world. It was used in a wide range of applications such as tires, hoses, jacketing for wires, the soles of shoes, and water-proof materials. Because rubber was required for so many common products, Thomas Edison and Henry Ford both had a hand in investigating possible domestic sources of NR, namely goldenrod and milkweed.2 Interest increased during World War II when the Allies’ access to rubber in Southeast Asia6 was severely cut off, and there were still no domestic sources. This posed a problem:
“A modern nation could not hope to defend itself without rubber. The construction of a military airplane used one-half ton of rubber; a tank needed about 1 ton and a battleship, 75 tons. Each person in the military required 32 pounds of rubber for footwear, clothing, and equipment. Tires were needed for all kinds of vehicles and aircraft.7”
However, acquiring rubber from milkweed or goldenrod was not feasible on a scale large enough to supply the war effort. The U.S. instead implemented the synthetic rubber program, a grand undertaking that ultimately succeeded and no doubt influenced the outcome of the war.7 With the advent of quality synthetic rubbers in the 1940s, the demand for a domestic source of NR was virtually eliminated until the oil shortages of the 1970s, when research into extraction of rubber from domestic plants began to resurge.3
Today, interest in obtaining commodity chemicals–such as ethanol for fuel use–from renewable sources has rebounded due to the prospect of declining oil availability and increasing levels of atmospheric carbon.8,9 In 2007, ca. 13% of the crude oil consumption in the US was used for non-fuel chemical production.4 Without the vast, inexpensive source of hydrocarbon byproducts of petroleum refinement, chemical industries may begin to rely more heavily on biological sources for their supply of commodity chemicals.8
One commodity chemical used to make synthetic rubber is isoprene. Isoprene was first isolated by the thermal depolymerization of NR in 1860, by C.G. Williams (Scheme 1.).10 Most attempts to depolymerize natural rubber yield low molecular weight liquid natural rubber (LNR).11,12,13 LNR chains are shorter, and typically terminated with oxygen bearing functional groups that increase the utility of the molecule and thus garnered more research focus.12 Ravindran and coworkers reported partial photocatalyzed radical degradation of natural rubber at room temperature in the presence of hydrogen peroxide to produce hydroxyl terminated LNR.12 Sakdapipanich and coworkers reported a metal-catalyzed oxidation depolymerization with Co(acac)2 yielding LNR.13 Inspired by these reports of partial depolymerization we report our attempts to develop a new, efficient synthetic strategy in order to extend the reaction to obtain isoprene from natural rubber.
Results and Discussion
The extraction of natural rubber from milkweed leaves by serial solid liquid extractions was effective and facile. Initially an aqueous extraction was used to remove the water soluble compounds at reflux for 30 min. Next an acetone extraction removed the terpenoids, isoprenoids, glycerols, and sterols.4 Finally a toluene extraction allowed for the recovery of polyisoprene from the milkweed leaves. The yield by mass was 2.2 % which is comparable to yields of 2.4 ± 1.8 % reported by Volaric4 but less than the 4.4 % reported by Harvey and Hallend.5 Recovery of NR was confirmed by 1H NMR and comparison to a known standard. Our method of extraction, though not as high yielding as others, was efficient and required no specialized equipment or difficult set ups.
A total of five depolymerization reactions were attempted to obtain isoprene (Table 1). Since the amount of NR extracted from milkweed was on the milligram scale, depolymerization experiments utilized NR acquired from commercial suppliers.Thermal depolymerization at 450 °C with a copper wire packed fractioning column, which ensured that short oligomers would not carry over with the distillate, successfully afforded isoprene in 2.2 % yield. The peroxide catalyst had no effect, evidenced by 1H NMR. Using a catalytic amount of Fe2(CO)10 produced a mixture of oligomers and a small amount of isoprene, evidenced by GC and 1H NMR while the reaction with Pd(PPh3)4 yielded a thick brown tar.
Reflux times were constrained to 4 h to ensure efficiency, so it is possible that the catalyzed reactions simply had not progressed to the point of producing any isoprene before the distillation. This could explain why oligomers, rather than isoprene, were predominantly isolated in the Fe2(CO)10 catalyzed reactions. However additional investigation will be required in order to identify an effective, low temperature depolymerization metal catalyst. Next we may focus on a Ziegler-Natta complex like TiCl4 that may work better than the peroxide and alkene coordinating complexes we surveyed.
Conclusion
A fast and simple method for the extraction of polyisoprene from A. syriaca leaves was developed. Fe2(CO)10 was the most promising catalyst surveyed, with oligomers and a small amount of isoprene observed in the distillate. Thermal decomposition of polyisoprene was successful in yielding measurable amounts of isoprene.
Experimental Section
General. Milkweed leaves were picked from mature plants at the Central College Field Station in late July, 2015. The leaves were packed in mason jars and stored frozen prior to use. The solvents used were reagent grade and obtained from Sigma-Aldrich. Pd(PPh3)4, Fe2(CO)10, di-tert-butylperoxide, and cis-polyisoprene were reagent grade and used without further purification. GC spectra were taken on a HP 6890 Series with a 30 m × 0.32 mm × 0.25 μm 5% crosslinked PH ME siloxane column. 1H NMR spectra were recorded on a 300 MHz spectrometer. Chemical shifts are expressed in parts per million (δ) using TMS as an internal standard.
Extraction of Polyisoprene From Milkweed. Six milkweed leaves were flash frozen in liquid nitrogen, then ground with a mortar and pestle. The resulting powder (17.05 g) and 150mL of DI water was transferred to a 250 mL Erlenmeyer flask. The solution was refluxed for 30 min, vacuum filtered, and the retentate washed with water and transferred to another 250 mL Erlenmeyer flask along with 150 mL acetone. The solution was then refluxed for 1.5 h, vacuum filtered, and the retentate washed with acetone until the filtrate was colorless. The resulting pale yellow powder was then transferred to a 250 mL Erlenmeyer flask with 100 mL toluene. The solution was refluxed for 1 h and then vacuum filtered. The filtrate was concentrated by heated evaporation under a stream of nitrogen affording 375 mg (2.2%) polyisoprene as a yellow white solid. 1H NMR (300 MHz, CDCl3) δ 5.300 (t, J = 6.0, 1H), 2.208 (m, 4H), 1.773 (s, 3H).
Thermal Decomposition of Polyisoprene. To a 50 mL round bottom flask was added 1.03 g polyisoprene. A reflux condenser packed with copper wire and a distillation head were fitted to the flask, and a short path distillation head was attached to the condenser. The entire set up was wrapped in cotton for insulation. An udder was attached to the distillation head and immersed in liquid nitrogen. The round bottom was heated in a sand bath at ca. 450 °C . Isoprenewas collected in 2% yield (20 mg) as a clear liquid. 1H NMR (300 MHz, CDCl3) δ 6.453 (dd, J = 21.9, 10.5, 1H), 5.124 (dd, J = 32.6, 10.5, 2H), 5.00 (d, J = 4.5, 2H), 1.850 (s, 3H).
Depolymerization with Peroxide. To a 10 mL round bottom flask was added 0.202 g cis-polyisoprene, 6 mL toluene, and 50 mg t-butylperoxide. A reflux condenser packed with copper wire and a distillation head were fitted to the flask, which was then immersed in a 125 °C oil bath. The solution was allowed to reflux for 2 h. The flow of water through the condenser was stopped and the solution was allowed to distill into an udder immersed in liquid nitrogen. After 3 h, no distillate was collected. The solution was allowed to cool, and concentrated by boiling under a stream of nitrogen. The resulting brown tar was characterized by 1H NMR and found to be polyisoprene.
Depolymerization with Fe2(CO)10. To a 10 mL round bottom flask was added 0.332 g cis-polyisoprene, 7 mL toluene, and 4 mg Fe2(CO)10. A reflux condenser packed with copper wire and a distillation head were fitted to the flask, which was then immersed in a 125 °C oil bath. The solution was allowed to reflux for 2 h. The solution was allowed to distill into an udder immersed in liquid nitrogen. Two drops of a light brown distillate were collected. GC data suggests the distillate was a mixture of oligomers of isoprene.
Depolymerization with Pd(PPh3)4. To a 10 mL round bottom flask was added 0.333 g cis-polyisoprene, 7 mL toluene, and 2 mg Pd(PPh3)4. A reflux condenser packed with copper wire and a distillation head were fitted to the flask, which was then immersed in a 125 °C oil bath. The solution was allowed to reflux for 2 h. After refluxing, the solution had turned to a dark brown liquid with solid dispersed throughout which was insoluble in all attempted solvents.
Works Cited
- Kauffman G.B.; Seymour, R.B. Elastomers I. Natural Rubber. J. Chem. Educ. 1990, 65, 422.
- Johnson, M. Edison, a Biography; McGraw Hill: New York, 1959; 470–474.
- Buchanan, R. A. Extraction of Rubber or Rubberlike Substances from Fibrous Plant Materials; U.S. Patent 4,136,131, 1978.
- Volaric, L.; Hagen, J. The Isolation of Rubber from Milkweed Leaves. J. Chem. Educ. 2002, 79, 91-93.
- Harvey, M.; Halland, L.F. Rubber-Content of North American Plants. Carnegie Institution of Washington 1921.
- Before 1880, rubber production was localized in South America, mainly Brazil. It was believed that rubber trees could not grow outside the Amazon basin. In 1876, Henry Wickham smuggled seventy thousand Hevea brasiliensis seeds from the Amazon to England. With the help of the botanist Joseph Hooker and the support of the Royal Botanical Gardens, intensive scientific study of the cultivation and tapping of the rubber tree began. By 1907, the British had established rubber plantations with a total of around ten million trees in Malaya and Ceylon and had effectively dominated the rubber trade localizing the major source of NR to Southeast Asia to this day. Le Counteur, P.; Burreson, J. Napoleon’s Buttons: 17 Molecules that Changed History; Jeremy P. Tarcher/Pengiun: New York, 2004; pp 152-154
- American Chemical Society National Historic Chemical Landmarks. U.S. Synthetic Rubber Program. http://www.acs.org/content/acs/en/education/whatischemistry/landmarks/syntheticrubber.html (accessed Sept 10, 2015).
- Dodds, D. R.; Gross, R. A. Chemicals from Biomass. Science, 2007, 318, 1250-1251.
- Campbell C.J.; Laherrere, J.H. The End of Cheap Oil. Sci. Am. 1998, 78-83.
- C. G. Williams, Proceedings of the Royal Society, 1860, 10.
- I. Suhawati, A. Mustafa. Chemical Depolymerisation of Natural Rubber in Biphasic Medium. Advanced Materials Research, 2014, 1024, 193-196.
- Ravindran, T.; Nayar, M.R.G.; Francis, D.F. Production of Hydroxyl-Terminated Liquid Natural Rubber. J. of Appl. Poly. Sci. 1988, 35, 1227-1239.
- Sakdapipanich, T.; Yasuyuki; et al. Depolymerisation of Highly Purified Natural Rubber. I. Metal-catalysed Oxidation of Deproteinised Natural Rubber. Journal of Rubber Research (Kuala Lumpur, Malaysia), 2001, 4, 1-10.